Systems Deep Dive
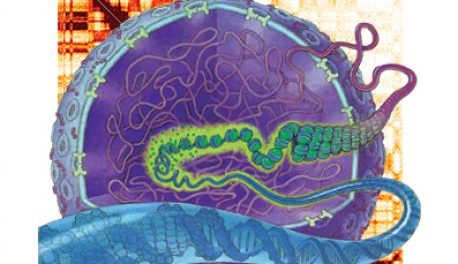
Rachel Patton McCord’s interest in science is in her genes. She learned how to be curious about the natural world and how it works from her parents, who were both scientists.
“I’ve always loved puzzles and solving problems,” says McCord, assistant professor in the Department of Biochemistry and Cellular & Molecular Biology (BCMB), who earned her PhD in biophysics from Harvard University and joined the UT faculty in 2016.
McCord is still solving problems, but the ones she works on now involve 3-D genome structures. In her lab, she focuses on measuring the 3-D interactions between all regions of DNA inside human cell nuclei.
“We start by considering the basic problem that the six-foot long human DNA sequence must fold into a microscopic space,” McCord says. “This compact folding must still allow it to do its basic functions of regulating genes at the right time, replicating itself before cell division, and repairing itself after damage.”
Most genetic analysis treats genes as isolated units along a linear string of DNA. The looping and folding of chromosomes, however, is essential to gene regulation, DNA repair, and properly coping and separating the DNA into two new cells during cell division. Loops in the DNA allow different parts of the genome to talk to each other. If these loops get disorganized, problems such as cancer or developmental defects can be the result.
“The unique piece we work on is how the 3-D structure of the genome and all the loops and domains it forms are affected in situations where there are major physical deformations of the nucleus,” McCord says. “We hope our research will shed light on how the physical reshaping of 3-D chromosome folding contributes to cancer metastasis and premature aging.”
For decades, scientists have studied complex and interconnected ecosystems or anatomical systems. The advent of new experimental techniques, such as the ones McCord uses in her lab, coupled with increasing computing power have allowed scientists to study systems biology at the molecular level.
In order to study the complex gene regulatory networks, scientists need mathematical models, which is the focus of Tian Hong’s work.
“The interactions of our genes are so complex that it is very hard to understand them intuitively,” says Hong, assistant professor in BCMB. “We need help from mathematical models to gain some insight into these gene regulatory networks. By doing this, we get closer to understanding the dynamics of cells and tissues and how diseases occur due to the misregulation of these networks.”
Hong focuses on building mathematical models to study epithelial plasticity and the immune system. The epithelial cell exists in the epidermis and tissues, such as mammary glands. The plasticity of the epithelial cell – its ability to take on characteristics of cells elsewhere in the body – is important because it is the basis of cancer metastasis.
“The onset of the spread of cancer is the transformation of these epithelial cells,” Hong says. “In order to move through the bloodstream, the cancer cells have to undergo a transformation. This type of cell is very migratory. Understanding how these cells start to migrate and transform once they arrive at the secondary organs will help us understand how they proliferate and form a new tumor.”
In the immune system, cells can quickly diversify when there is an infection or pathogen. If there is an excessive response, however, tissues will be damaged.
“We need to protect our body by having some of the more aggressive types of immune cells to fight against the pathogens, but we have to balance the type of immune response,” Hong says. “Some cells will be responsible to fight the pathogens and others will ensure the immune response can be attenuated so that our own tissues do not get damaged.”
Mathematical models will allow Hong to study the immune system and the transformation of the epithelial cell to gain a better understanding of these complex systems. Once scientists understand how these systems work and how they interact with the human body, they will be able to understand and fight diseases that attack these networks.
Systems biology requires significant computing power. The supercomputers owned and operated by UT and Oak Ridge National Laboratory computational scientists offer BCMB faculty and students an unrivaled computing power in the form of TITAN, the fourth most powerful supercomputer in the world.
With such computing power at their fingertips, BCMB faculty and students are on the cutting edge in teaching and research in computational sciences and systems biology.
Leave a comment